图书介绍
molecular biology of the cellpdf电子书版本下载
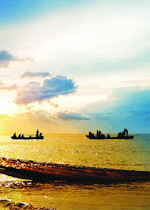
- 著
- 出版社:
- ISBN:
- 出版时间:未知
- 标注页数:0页
- 文件大小:328MB
- 文件页数:1464页
- 主题词:
PDF下载
下载说明
molecular biology of the cellPDF格式电子书版下载
下载的文件为RAR压缩包。需要使用解压软件进行解压得到PDF格式图书。建议使用BT下载工具Free Download Manager进行下载,简称FDM(免费,没有广告,支持多平台)。本站资源全部打包为BT种子。所以需要使用专业的BT下载软件进行下载。如 BitComet qBittorrent uTorrent等BT下载工具。迅雷目前由于本站不是热门资源。不推荐使用!后期资源热门了。安装了迅雷也可以迅雷进行下载!
(文件页数 要大于 标注页数,上中下等多册电子书除外)
注意:本站所有压缩包均有解压码: 点击下载压缩包解压工具
图书目录
Chapter 1 Cells and Genomes 1
THE UNIVERSAL FEATURES OF CELLS ON EARTH 2
All Cells Store Their Hereditary Information in the Same Linear Chemical Code: DNA 2
All Cells Replicate Their Hereditary Information by Templated Polymerization 3
All Cells Transcribe Portions of Their Hereditary Information into the Same Intermediary Form: RNA 4
All Cells Use Proteins as Catalysts 5
All Cells Translate RNA into Protein in the Same Way 6
Each Protein Is Encoded by a Specific Gene 7
Life Requires Free Energy 8
All Cells Function as Biochemical Factories Dealing with the Same Basic Molecular Building Blocks 8
All Cells Are Enclosed in a Plasma Membrane Across Which Nutrients and Waste Materials Must Pass 8
A Living Cell Can Exist with Fewer Than 500 Genes 9
Summary 10
THE DIVERSITY OF GENOMES AND THE TREE OF LIFE 10
Cells Can Be Powered by a Variety of Free-Energy Sources 10
Some Cells Fix Nitrogen and Carbon Dioxide for Others 12
The Greatest Biochemical Diversity Exists Among Prokaryotic Cells 12
The Tree of Life Has Three Primary Branches: Bacteria, Archaea,and Eukaryotes 14
Some Genes Evolve Rapidly; Others Are Highly Conserved 15
Most Bacteria and Archaea Have 1000-6000 Genes 16
New Genes Are Generated from Preexisting Genes 16
Gene Duplications Give Rise to Families of Related Genes Within a Single Cell 17
Genes Can Be Transferred Between Organisms, Both in the Laboratory and in Nature 18
Sex Results in Horizontal Exchanges of Genetic Information Within a Species 19
The Function of a Gene Can Often Be Deduced from Its Sequence 20
More Than 200 Gene Families Are Common to All Three Primary Branches of the Tree of Life 20
Mutations Reveal the Functions of Genes 21
Molecular Biology Began with a Spotlight on E. coli 22
Summary 22
GENETIC INFORMATION IN EUKARYOTES 23
Eukaryotic Cells May Have Originated as Predators 24
Modern Eukaryotic Cells Evolved from a Symbiosis 25
Eukaryotes Have Hybrid Genomes 27
Eukaryotic Genomes Are Big 28
Eukaryotic Genomes Are Rich in Regulatory DNA 29
The Genome Defines the Program of Multicellular Development 29
Many Eukaryotes Live as Solitary Cells 30
A Yeast Serves as a Minimal Model Eukaryote 30
The Expression Levels of All the Genes of An Organism Can Be Monitored Simultaneously 32
Arabidopsis Has Been Chosen Out of 300,000 Species As a Model Plant 32
The World of Animal Cells Is Represented By a Worm, a Fly,a Fish, a Mouse, and a Human 33
Studies in Drosophila Provide a Key to Vertebrate Development 33
The Vertebrate Genome Is a Product of Repeated Duplications 34
The Frog and the Zebrafish Provide Accessible Models for Vertebrate Development 35
The Mouse Is the Predominant Mammalian Model Organism 35
Humans Report on Their Own Peculiarities 36
We Are All Different in Detail 38
To Understand Cells and Organisms Will Require Mathematics,Computers, and Quantitative Information 38
Summary 39
Problems 39
References 41
Chapter 2 Cell Chemistry and Bioenergetics 43
THE CHEMICAL COMPONENTS OF A CELL 43
Water Is Held Together by Hydrogen Bonds 44
Four Types of Noncovalent Attractions Help Bring Molecules Together in Cells 44
Some Polar Molecules Form Acids and Bases in Water 45
A Cell Is Formed from Carbon Compounds 47
Cells Contain Four Major Families of Small Organic Molecules 47
The Chemistry of Cells Is Dominated by Macromolecules with Remarkable Properties 47
Noncovalent Bonds Specify Both the Precise Shape of a Macromolecule and Its Binding to Other Molecules 49
Summary 50
CATALYSIS AND THE USE OF ENERGY BY CELLS 51
Cell Metabolism Is Organized by Enzymes 51
Biological Order Is Made Possible by the Release of Heat Energy from Cells 52
Cells Obtain Energy by the Oxidation of Organic Molecules 54
Oxidation and Reduction Involve Electron Transfers 55
Enzymes Lower the Activation-Energy Barriers That Block Chemical Reactions 57
Enzymes Can Drive Substrate Molecules Along Specific Reaction Pathways 58
How Enzymes Find Their Substrates: The Enormous Rapidity of Molecular Motions 59
The Free-Energy Change for a Reaction, △G, Determines Whether It Can Occur Spontaneously 60
The Concentration of Reactants Influences the Free-Energy Change and a Reaction's Direction 61
The Standard Free-Energy Change, △G°, Makes It Possible to Compare the Energetics of Different Reactions 61
The Equilibrium Constant and △G° Are Readily Derived from Each Other 62
The Free-Energy Changes of Coupled Reactions Are Additive 63
Activated Carrier Molecules Are Essential for Biosynthesis 63
The Formation of an Activated Carrier Is Coupled to an Energetically Favorable Reaction 64
ATP Is the Most Widely Used Activated Carrier Molecule 65
Energy Stored in ATP Is Often Harnessed to Join Two Molecules Together 65
NADH and NADPH Are Important Electron Carriers 67
There Are Many Other Activated Carrier Molecules in Cells 68
The Synthesis of Biological Polymers Is Driven by ATP Hydrolysis 70
Summary 73
HOW CELLS OBTAIN ENERGY FROM FOOD 73
Glycolysis Is a Central ATP-Producing Pathway 74
Fermentations Produce ATP in the Absence of Oxygen 75
Glycolysis Illustrates How Enzymes Couple Oxidation to Energy Storage 76
Organisms Store Food Molecules in Special Reservoirs 78
Most Animal Cells Derive Their Energy from Fatty Acids Between Meals 81
Sugars and Fats Are Both Degraded to Acetyl CoA in Mitochondria 81
The Citric Acid Cycle Generates NADH by Oxidizing Acetyl Groups to CO2 82
Electron Transport Drives the Synthesis of the Majority of the ATP in Most Cells 84
Amino Acids and Nucleotides Are Part of the Nitrogen Cycle 85
Metabolism Is Highly Organized and Regulated 87
Summary 88
Problems 88
References 108
Chapter 3 Proteins 109
THE SHAPE AND STRUCTURE OF PROTEINS 109
The Shape of a Protein Is Specified by Its Amino Acid Sequence 109
Proteins Fold into a Conformation of Lowest Energy 114
The α Helix and the β Sheet Are Common Folding Patterns 115
Protein Domains Are Modular Units from Which Larger Proteins Are Built 117
Few of the Many Possible Polypeptide Chains Will Be Useful to Cells 118
Proteins Can Be Classified into Many Families 119
Some Protein Domains Are Found in Many Different Proteins 121
Certain Pairs of Domains Are Found Together in Many Proteins 122
The Human Genome Encodes a Complex Set of Proteins,Revealing That Much Remains Unknown 122
Larger Protein Molecules Often Contain More Than One Polypeptide Chain 123
Some Globular Proteins Form Long Helical Filaments 123
Many Protein Molecules Have Elongated, Fibrous Shapes 124
Proteins Contain a Surprisingly Large Amount of Intrinsically Disordered Polypeptide Chain 125
Covalent Cross-Linkages Stabilize Extracellular Proteins 127
Protein Molecules Often Serve as Subunits for the Assembly of Large Structures 127
Many Structures in Cells Are Capable of Self-Assembly 128
Assembly Factors Often Aid the Formation of Complex Biological Structures 130
Amyloid Fibrils Can Form from Many Proteins 130
Amyloid Structures Can Perform Useful Functions in Cells 132
Many Proteins Contain Low-complexity Domains that Can Form “Reversible Amyloids” 132
Summary 134
PROTEIN FUNCTION 134
All Proteins Bind to Other Molecules 134
The Surface Conformation of a Protein Determines Its Chemistry 135
Sequence Comparisons Between Protein Family Members Highlight Crucial Ligand-Binding Sites 136
Proteins Bind to Other Proteins Through Several Types of Interfaces 137
Antibody Binding Sites Are Especially Versatile 138
The Equilibrium Constant Measures Binding Strength 138
Enzymes Are Powerful and Highly Specific Catalysts 140
Substrate Binding Is the First Step in Enzyme Catalysis 141
Enzymes Speed Reactions by Selectively Stabilizing Transition States 141
Enzymes Can Use Simultaneous Acid and Base Catalysis 144
Lysozyme Illustrates How an Enzyme Works 144
Tightly Bound Small Molecules Add Extra Functions to Proteins 146
Multienzyme Complexes Help to Increase the Rate of Cell Metabolism 148
The Cell Regulates the Catalytic Activities of Its Enzymes 149
Allosteric Enzymes Have Two or More Binding Sites That Interact 151
Two Ligands Whose Binding Sites Are Coupled Must Reciprocally Affect Each Other's Binding 151
Symmetric Protein Assemblies Produce Cooperative Allosteric Transitions 152
Many Changes in Proteins Are Driven by Protein Phosphorylation 153
A Eukaryotic Cell Contains a Large Collection of Protein Kinases and Protein Phosphatases 154
The Regulation of the Src Protein Kinase Reveals How a Protein Can Function as a Microprocessor 155
Proteins That Bind and Hydrolyze GTP Are Ubiquitous Cell Regulators 156
Regulatory Proteins GAP and GEF Control the Activity of GTP-Binding Proteins by Determining Whether GTP or GDP Is Bound 157
Proteins Can Be Regulated by the Covalent Addition of Other Proteins 157
An Elaborate Ubiquitin-Conjugating System Is Used to Mark Proteins 158
Protein Complexes with Interchangeable Parts Make Efficient Use of Genetic Information 159
A GTP-Binding Protein Shows How Large Protein Movements Can Be Generated 160
Motor Proteins Produce Large Movements in Cells 161
Membrane-Bound Transporters Harness Energy to Pump Molecules Through Membranes 163
Proteins Often Form Large Complexes That Function as Protein Machines 164
Scaffolds Concentrate Sets of Interacting Proteins 164
Many Proteins Are Controlled by Covalent Modifications That Direct Them to Specific Sites Inside the Cell 165
A Complex Network of Protein Interactions Underlies Cell Function 166
Summary 169
Problems 170
References 172
Chapter 4 DNA, Chromosomes, and Genomes 175
THE STRUCTURE AND FUNCTION OF DNA 175
A DNA Molecule Consists of Two Complementary Chains of Nucleotides 175
The Structure of DNA Provides a Mechanism for Heredity 177
In Eukaryotes, DNA Is Enclosed in a Cell Nucleus 178
Summary 179
CHROMOSOMAL DNA AND ITS PACKAGING IN THE CHROMATIN FIBER 179
Eukaryotic DNA Is Packaged into a Set of Chromosomes 180
Chromosomes Contain Long Strings of Genes 182
The Nucleotide Sequence of the Human Genome Shows How Our Genes Are Arranged 183
Each DNA Molecule That Forms a Linear Chromosome Must Contain a Centromere, Two Telomeres, and Replication Origins 185
DNA Molecules Are Highly Condensed in Chromosomes 187
Nucleosomes Are a Basic Unit of Eukaryotic Chromosome Structure 187
The Structure of the Nucleosome Core Particle Reveals How DNA Is Packaged 188
Nucleosomes Have a Dynamic Structure, and Are Frequently Subjected to Changes Catalyzed by ATP-Dependent Chromatin Remodeling Complexes 190
Nucleosomes Are Usually Packed Together into a Compact Chromatin Fiber 191
Summary 193
CHROMATIN STRUCTURE AND FUNCTION 194
Heterochromatin Is Highly Organized and Restricts Gene Expression 194
The Heterochromatic State Is Self-Propagating 194
The Core Histones Are Covalently Modified at Many Different Sites 196
Chromatin Acquires Additional Variety Through the Site-Specific Insertion of a Small Set of Histone Variants 198
Covalent Modifications and Histone Variants Act in Concert to Control Chromosome Functions 198
A Complex of Reader and Writer Proteins Can Spread Specific Chromatin Modifications Along a Chromosome 199
Barrier DNA Sequences Block the Spread of Reader-Writer Complexes and thereby Separate Neighboring Chromatin Domains 202
The Chromatin in Centromeres Reveals How Histone Variants Can Create Special Structures 203
Some Chromatin Structures Can Be Directly Inherited 204
Experiments with Frog Embryos Suggest that both Activating and Repressive Chromatin Structures Can Be Inherited Epigenetically 205
Chromatin Structures Are Important for Eukaryotic Chromosome Function 206
Summary 207
THE GLOBAL STRUCTURE OF CHROMOSOMES 207
Chromosomes Are Folded into Large Loops of Chromatin 207
Polytene Chromosomes Are Uniquely Useful for Visualizing Chromatin Structures 208
There Are Multiple Forms of Chromatin 210
Chromatin Loops Decondense When the Genes Within Them Are Expressed 211
Chromatin Can Move to Specific Sites Within the Nucleus to Alter Gene Expression 212
Networks of Macromolecules Form a Set of Distinct Biochemical Environments inside the Nucleus 213
Mitotic Chromosomes Are Especially Highly Condensed 214
Summary 216
HOW GENOMES EVOLVE 216
Genome Comparisons Reveal Functional DNA Sequences by their Conservation Throughout Evolution 217
Genome Alterations Are Caused by Failures of the Normal Mechanisms for Copying and Maintaining DNA, as well as by Transposable DNA Elements 217
The Genome Sequences of Two Species Differ in Proportion to the Length of Time Since They Have Separately Evolved 218
Phylogenetic Trees Constructed from a Comparison of DNA Sequences Trace the Relationships of All Organisms 219
A Comparison of Human and Mouse Chromosomes Shows How the Structures of Genomes Diverge 221
The Size of a Vertebrate Genome Reflects the Relative Rates of DNA Addition and DNA Loss in a Lineage 222
We Can Infer the Sequence of Some Ancient Genomes 223
Multispecies Sequence Comparisons Identify Conserved DNA Sequences of Unknown Function 224
Changes in Previously Conserved Sequences Can Help Decipher Critical Steps in Evolution 226
Mutations in the DNA Sequences That Control Gene Expression Have Driven Many of the Evolutionary Changes in Vertebrates 227
Gene Duplication Also Provides an Important Source of Genetic Novelty During Evolution 227
Duplicated Genes Diverge 228
The Evolution of the Globin Gene Family Shows How DNA Duplications Contribute to the Evolution of Organisms 229
Genes Encoding New Proteins Can Be Created by the Recombination of Exons 230
Neutral Mutations Often Spread to Become Fixed in a Population,with a Probability That Depends on Population Size 230
A Great Deal Can Be Learned from Analyses of the Variation Among Humans 232
Summary 234
Problems 234
References 236
Chapter 5 DNA Replication, Repair, and Recombination 237
THE MAINTENANCE OF DNA SEQUENCES 237
Mutation Rates Are Extremely Low 237
Low Mutation Rates Are Necessary for Life as We Know It 238
Summary 239
DNA REPLICATION MECHANISMS 239
Base-Pairing Underlies DNA Replication and DNA Repair 239
The DNA Replication Fork Is Asymmetrical 240
The High Fidelity of DNA Replication Requires Several Proofreading Mechanisms 242
Only DNA Replication in the 5′-to-3′ Direction Allows Efficient Error Correction 244
A Special Nucleotide-Polymerizing Enzyme Synthesizes Short RNA Primer Molecules on the Lagging Strand 245
Special Proteins Help to Open Up the DNA Double Helix in Front of the Replication Fork 246
A Sliding Ring Holds a Moving DNA Polymerase Onto the DNA 246
The Proteins at a Replication Fork Cooperate to Form a Replication Machine 249
A Strand-Directed Mismatch Repair System Removes Replication Errors That Escape from the Replication Machine 250
DNA Topoisomerases Prevent DNA Tangling During Replication 251
DNA Replication Is Fundamentally Similar in Eukaryotes and Bacteria 253
Summary 254
THE INITIATION AND COMPLETION OF DNA REPLICATION IN CHROMOSOMES 254
DNA Synthesis Begins at Replication Origins 254
Bacterial Chromosomes Typically Have a Single Origin of DNA Replication 255
Eukaryotic Chromosomes Contain Multiple Origins of Replication 256
In Eukaryotes, DNA Replication Takes Place During Only One Part of the Cell Cycle 258
Different Regions on the Same Chromosome Replicate at Distinct Times in S Phase 258
A Large Multisubunit Complex Binds to Eukaryotic Origins of Replication 259
Features of the Human Genome That Specify Origins of Replication Remain to Be Discovered 260
New Nucleosomes Are Assembled Behind the Replication Fork 261
Telomerase Replicates the Ends of Chromosomes 262
Telomeres Are Packaged Into Specialized Structures That Protect the Ends of Chromosomes 263
Telomere Length Is Regulated by Cells and Organisms 264
Summary 265
DNA REPAIR 266
Without DNA Repair, Spontaneous DNA Damage Would Rapidly Change DNA Sequences 267
The DNA Double Helix Is Readily Repaired 268
DNA Damage Can Be Removed by More Than One Pathway 269
Coupling Nucleotide Excision Repair to Transcription Ensures That the Cell's Most Important DNA Is Efficiently Repaired 271
The Chemistry of the DNA Bases Facilitates Damage Detection 271
Special Translesion DNA Polymerases Are Used in Emergencies 273
Double-Strand Breaks Are Efficiently Repaired 273
DNA Damage Delays Progression of the Cell Cycle 276
Summary 276
HOMOLOGOUS RECOMBINATION 276
Homologous Recombination Has Common Features in All Cells 277
DNA Base-Pairing Guides Homologous Recombination 277
Homologous Recombination Can Flawlessly Repair Double-Strand Breaks in DNA 278
Strand Exchange Is Carried Out by the RecA/Rad51 Protein 279
Homologous Recombination Can Rescue Broken DNA Replication Forks 280
Cells Carefully Regulate the Use of Homologous Recombination in DNA Repair 280
Homologous Recombination Is Crucial for Meiosis 282
Meiotic Recombination Begins with a Programmed Double-Strand Break 282
Holliday Junctions Are Formed During Meiosis 284
Homologous Recombination Produces Both Crossovers and Non-Crossovers During Meiosis 284
Homologous Recombination Often Results in Gene Conversion 286
Summary 286
TRANSPOSITION AND CONSERVATIVE SITE-SPECIFIC RECOMBINATION 287
Through Transposition, Mobile Genetic Elements Can Insert Into Any DNA Sequence 288
DNA-Only Transposons Can Move by a Cut-and-Paste Mechanism 288
Some Viruses Use a Transposition Mechanism to Move Themselves Into Host-Cell Chromosomes 290
Retroviral-like Retrotransposons Resemble Retroviruses, but Lack a Protein Coat 291
A Large Fraction of the Human Genome Is Composed of Nonretroviral Retrotransposons 291
Different Transposable Elements Predominate in Different Organisms 292
Genome Sequences Reveal the Approximate Times at Which Transposable Elements Have Moved 292
Conservative Site-Specific Recombination Can Reversibly Rearrange DNA 292
Conservative Site-Specific Recombination Can Be Used to Turn Genes On or Off 294
Bacterial Conservative Site-Specific Recombinases Have Become Powerful Tools for Cell and Developmental Biologists 294
Summary 295
Problems 296
References 298
Chapter 6 How Cells Read the Genome:From DNA to Protein 299
FROM DNA TO RNA 301
RNA Molecules Are Single-Stranded 302
Transcription Produces RNA Complementary to One Strand of DNA 302
RNA Polymerases Carry Out Transcription 303
Cells Produce Different Categories of RNA Molecules 305
Signals Encoded in DNA Tell RNA Polymerase Where to Start and Stop 306
Transcription Start and Stop Signals Are Heterogeneous in Nucleotide Sequence 307
Transcription Initiation in Eukaryotes Requires Many Proteins 309
RNA Polymerase II Requires a Set of General Transcription Factors 310
Polymerase II Also Requires Activator, Mediator, and Chromatin-Modifying Proteins 312
Transcription Elongation in Eukaryotes Requires Accessory Proteins 313
Transcription Creates Superhelical Tension 314
Transcription Elongation in Eukaryotes Is Tightly Coupled to RNA Processing 315
RNA Capping Is the First Modification of Eukaryotic Pre-mRNAs 316
RNA Splicing Removes Intron Sequences from Newly Transcribed Pre-mRNAs 317
Nucleotide Sequences Signal Where Splicing Occurs 319
RNA Splicing Is Performed by the Spliceosome 319
The Spliceosome Uses ATP Hydrolysis to Produce a Complex Series of RNA-RNA Rearrangements 321
Other Properties of Pre-mRNA and Its Synthesis Help to Explain the Choice of Proper Splice Sites 321
Chromatin Structure Affects RNA Splicing 323
RNA Splicing Shows Remarkable Plasticity 323
Spliceosome-Catalyzed RNA Splicing Probably Evolved from Self-splicing Mechanisms 324
RNA-Processing Enzymes Generate the 3′ End of Eukaryotic mRNAs 324
Mature Eukaryotic mRNAs Are Selectively Exported from the Nucleus 325
Noncoding RNAs Are Also Synthesized and Processed in the Nucleus 327
The Nucleolus Is a Ribosome-Producing Factory 329
The Nucleus Contains a Variety of Subnuclear Aggregates 331
Summary 333
FROM RNA TO PROTEIN 333
An mRNA Sequence Is Decoded in Sets of Three Nucleotides 334
tRNA Molecules Match Amino Acids to Codons in mRNA 334
tRNAs Are Covalently Modified Before They Exit from the Nucleus 336
Specific Enzymes Couple Each Amino Acid to Its Appropriate tRNA Molecule 336
Editing by tRNA Synthetases Ensures Accuracy 338
Amino Acids Are Added to the C-terminal End of a Growing Polypeptide Chain 339
The RNA Message Is Decoded in Ribosomes 340
Elongation Factors Drive Translation Forward and Improve Its Accuracy 343
Many Biological Processes Overcome the Inherent Limitations of Complementary Base-Pairing 345
Accuracy in Translation Requires an Expenditure of Free Energy 345
The Ribosome Is a Ribozyme 346
Nucleotide Sequences in mRNA Signal Where to Start Protein Synthesis 347
Stop Codons Mark the End of Translation 348
Proteins Are Made on Polyribosomes 349
There Are Minor Variations in the Standard Genetic Code 349
Inhibitors of Prokaryotic Protein Synthesis Are Useful as Antibiotics 351
Quality Control Mechanisms Act to Prevent Translation of Damaged mRNAs 351
Some Proteins Begin to Fold While Still Being Synthesized 353
Molecular Chaperones Help Guide the Folding of Most Proteins 354
Cells Utilize Several Types of Chaperones 355
Exposed Hydrophobic Regions Provide Critical Signals for Protein Quality Control 357
The Proteasome Is a Compartmentalized Protease with Sequestered Active Sites 357
Many Proteins Are Controlled by Regulated Destruction 359
There Are Many Steps From DNA to Protein 361
Summary 362
THE RNA WORLD AND THE ORIGINS OF LIFE 362
Single-Stranded RNA Molecules Can Fold into Highly Elaborate Structures 363
RNA Can Both Store Information and Catalyze Chemical Reactions 364
How Did Protein Synthesis Evolve? 365
All Present-Day Cells Use DNA as Their Hereditary Material 365
Summary 366
Problems 366
References 368
Chapter 7 Control of Gene Expression 369
AN OVERVIEW OF GENE CONTROL 369
The Different Cell Types of a Multicellular Organism Contain the Same DNA 369
Different Cell Types Synthesize Different Sets of RNAs and Proteins 370
External Signals Can Cause a Cell to Change the Expression of Its Genes 372
Gene Expression Can Be Regulated at Many of the Steps in the Pathway from DNA to RNA to Protein 372
Summary 373
CONTROL OF TRANSCRIPTION BY SEQUENCE-SPECIFIC DNA-BINDING PROTEINS 373
The Sequence of Nucleotides in the DNA Double Helix Can Be Read by Proteins 373
Transcription Regulators Contain Structural Motifs That Can Read DNA Sequences 374
Dimerization of Transcription Regulators Increases Their Affinity and Specificity for DNA 375
Transcription Regulators Bind Cooperatively to DNA 378
Nucleosome Structure Promotes Cooperative Binding of Transcription Regulators 379
Summary 380
TRANSCRIPTION REGULATORS SWITCH GENES ON AND OFF 380
The Tryptophan Repressor Switches Genes Off 380
Repressors Turn Genes Off and Activators Turn Them On 381
An Activator and a Repressor Control the Lac Operon 382
DNA Looping Can Occur During Bacterial Gene Regulation 383
Complex Switches Control Gene Transcription in Eukaryotes 384
A Eukaryotic Gene Control Region Consists of a Promoter Plus Many cis-Regulatory Sequences 384
Eukaryotic Transcription Regulators Work in Groups 385
Activator Proteins Promote the Assembly of RNA Polymerase at the Start Point of Transcription 386
Eukaryotic Transcription Activators Direct the Modification of Local Chromatin Structure 386
Transcription Activators Can Promote Transcription by Releasing RNA Polymerase from Promoters 388
Transcription Activators Work Synergistically 388
Eukaryotic Transcription Repressors Can Inhibit Transcription in Several Ways 389
Insulator DNA Sequences Prevent Eukaryotic Transcription Regulators from Influencing Distant Genes 391
Summary 392
MOLECULAR GENETIC MECHANISMS THAT CREATE AND MAINTAIN SPECIALIZED CELL TYPES 392
Complex Genetic Switches That Regulate Drosophilate Development Are Built Up from Smaller Molecules 392
The Drosophila Eve Gene Is Regulated by Combinatorial Controls 394
Transcription Regulators Are Brought Into Play by Extracellular Signals 395
Combinatorial Gene Control Creates Many Different Cell Types 396
Specialized Cell Types Can Be Experimentally Reprogrammed to Become Pluripotent Stem Cells 398
Combinations of Master Transcription Regulators Specify Cell Types by Controlling the Expression of Many Genes 398
Specialized Cells Must Rapidly Turn Sets of Genes On and Off 399
Differentiated Cells Maintain Their Identity 400
Transcription Circuits Allow the Cell to Carry Out Logic Operations 402
Summary 404
MECHANISMS THAT REINFORCE CELL MEMORY IN PLANTS AND ANIMALS 404
Patterns of DNA Methylation Can Be Inherited When Vertebrate Cells Divide 404
CG-Rich Islands Are Associated with Many Genes in Mammals 405
Genomic Imprinting Is Based on DNA Methylation 407
Chromosome-Wide Alterations in Chromatin Structure Can Be Inherited 409
Epigenetic Mechanisms Ensure That Stable Patterns of Gene Expression Can Be Transmitted to Daughter Cells 411
Summary 413
POST-TRANSCRIPTIONAL CONTROLS 413
Transcription Attenuation Causes the Premature Termination of Some RNA Molecules 414
Riboswitches Probably Represent Ancient Forms of Gene Control 414
Alternative RNA Splicing Can Produce Different Forms of a Protein from the Same Gene 415
The Definition of a Gene Has Been Modified Since the Discovery of Alternative RNA Splicing 416
A Change in the Site of RNA Transcript Cleavage and Poly-A Addition Can Change the C-terminus of a Protein 417
RNA Editing Can Change the Meaning of the RNA Message 418
RNA Transport from the Nucleus Can Be Regulated 419
Some mRNAs Are Localized to Specific Regions of the Cytosol 421
The 5′ and 3′ Untranslated Regions of mRNAs Control Their Translation 422
The Phosphorylation of an Initiation Factor Regulates Protein Synthesis Globally 423
Initiation at AUG Codons Upstream of the Translation Start Can Regulate Eukaryotic Translation Initiation 424
Internal Ribosome Entry Sites Provide Opportunities for Translational Control 425
Changes in mRNA Stability Can Regulate Gene Expression 426
Regulation of mRNA Stability Involves P-bodies and Stress Granules 427
Summary 428
REGULATION OF GENE EXPRESSION BY NONCODING RNAs 429
Small Noncoding RNA Transcripts Regulate Many Animal and Plant Genes Through RNA Interference 429
miRNAs Regulate mRNA Translation and Stability 429
RNA Interference Is Also Used as a Cell Defense Mechanism 431
RNA Interference Can Direct Heterochromatin Formation 432
piRNAs Protect the Germ Line from Transposable Elements 433
RNA Interference Has Become a Powerful Experimental Tool 433
Bacteria Use Small Noncoding RNAs to Protect Themselves from Viruses 433
Long Noncoding RNAs Have Diverse Functions in the Cell 435
Summary 436
Problems 436
References 438
Chapter 8 Analyzing Cells, Molecules, and Systems 439
ISOLATING CELLS AND GROWING THEM IN CULTURE 440
Cells Can Be Isolated from Tissues 440
Cells Can Be Grown in Culture 440
Eukaryotic Cell Lines Are a Widely Used Source of Homogeneous Cells 442
Hybridoma Cell Lines Are Factories That Produce Monoclonal Antibodies 444
Summary 445
PURIFYING PROTEINS 445
Cells Can Be Separated into Their Component Fractions 445
Cell Extracts Provide Accessible Systems to Study Cell Functions 447
Proteins Can Be Separated by Chromatography 448
Immunoprecipitation Is a Rapid Affinity Purification Method 449
Genetically Engineered Tags Provide an Easy Way to Purify Proteins 450
Purified Cell-free Systems Are Required for the Precise Dissection of Molecular Functions 451
Summary 451
ANALYZING PROTEINS 452
Proteins Can Be Separated by SDS Polyacrylamide-Gel Electrophoresis 452
Two-Dimensional Gel Electrophoresis Provides Greater Protein Separation 452
Specific Proteins Can Be Detected by Blotting with Antibodies 454
Hydrodynamic Measurements Reveal the Size and Shape of a Protein Complex 455
Mass Spectrometry Provides a Highly Sensitive Method for Identifying Unknown Proteins 455
Sets of Interacting Proteins Can Be Identified by Biochemical Methods 457
Optical Methods Can Monitor Protein Interactions 458
Protein Function Can Be Selectively Disrupted With Small Molecules 459
Protein Structure Can Be Determined Using X-Ray Diffraction 460
NMR Can Be Used to Determine Protein Structure in Solution 461
Protein Sequence and Structure Provide Clues About Protein Function 462
Summary 463
ANALYZING AND MANIPULATING DNA 463
Restriction Nucleases Cut Large DNA Molecules into Specific Fragments 464
Gel Electrophoresis Separates DNA Molecules of Different Sizes 465
Purified DNA Molecules Can Be Specifically Labeled with Radioisotopes or Chemical Markers in vitro 467
Genes Can Be Cloned Using Bacteria 467
An Entire Genome Can Be Represented in a DNA Library 469
Genomic and cDNA Libraries Have Different Advantages and Drawbacks 471
Hybridization Provides a Powerful, But Simple Way to Detect Specific Nucleotide Sequences 472
Genes Can Be Cloned in vitro Using PCR 473
PCR Is Also Used for Diagnostic and Forensic Applications 474
Both DNA and RNA Can Be Rapidly Sequenced 477
To Be Useful, Genome Sequences Must Be Annotated 477
DNA Cloning Allows Any Protein to be Produced in Large Amounts 483
Summary 484
STUDYING GENE EXPRESSION AND FUNCTION 485
Classical Genetics Begins by Disrupting a Cell Process by Random Mutagenesis 485
Genetic Screens Identify Mutants with Specific Abnormalities 488
Mutations Can Cause Loss or Gain of Protein Function 489
Complementation Tests Reveal Whether Two Mutations Are in the Same Gene or Different Genes 490
Gene Products Can Be Ordered in Pathways by Epistasis Analysis 490
Mutations Responsible for a Phenotype Can Be Identified Through DNA Analysis 491
Rapid and Cheap DNA Sequencing Has Revolutionized Human Genetic Studies 491
Linked Blocks of Polymorphisms Have Been Passed Down from Our Ancestors 492
Polymorphisms Can Aid the Search for Mutations Associated with Disease 493
Genomics Is Accelerating the Discovery of Rare Mutations That Predispose Us to Serious Disease 493
Reverse Genetics Begins with a Known Gene and Determines Which Cell Processes Require Its Function 494
Animals and Plants Can Be Genetically Altered 495
The Bacterial CRISPR System Has Been Adapted to Edit Genomes in a Wide Variety of Species 497
Large Collections of Engineered Mutations Provide a Tool for Examining the Function of Every Gene in an Organism 498
RNA Interference Is a Simple and Rapid Way to Test Gene Function 499
Reporter Genes Reveal When and Where a Gene Is Expressed 501
In situ Hybridization Can Reveal the Location of mRNAs and Noncoding RNAs 502
Expression of Individual Genes Can Be Measured Using Quantitative RT PCR 502
Analysis of mRNAs by Microarray or RNA-seq Provides a Snapshot of Gene Expression 503
Genome-wide Chromatin Immunoprecipitation Identifies Sites on the Genome Occupied by Transcription Regulators 505
Ribosome Profiling Reveals Which mRNAs Are Being Translated in the Cell 505
Recombinant DNA Methods Have Revolutionized Human Health 506
Transgenic Plants Are Important for Agriculture 507
Summary 508
MATHEMATICAL ANALYSIS OF CELL FUNCTIONS 509
Regulatory Networks Depend on Molecular Interactions 509
Differential Equations Help Us Predict Transient Behavior 512
Both Promoter Activity and Protein Degradation Affect the Rate of Change of Protein Concentration 513
The Time Required to Reach Steady State Depends on Protein Lifetime 514
Quantitative Methods Are Similar for Transcription Repressors and Activators 514
Negative Feedback Is a Powerful Strategy in Cell Regulation 515
Delayed Negative Feedback Can Induce Oscillations 516
DNA Binding By a Repressor or an Activator Can Be Cooperative 516
Positive Feedback Is Important for Switchlike Responses and Bistability 518
Robustness Is an Important Characteristic of Biological Networks 520
Two Transcription Regulators That Bind to the Same Gene Promoter Can Exert Combinatorial Control 520
An Incoherent Feed-forward Interaction Generates Pulses 522
A Coherent Feed-forward Interaction Detects Persistent Inputs 522
The Same Network Can Behave Differently in Different Cells Due to Stochastic Effects 523
Several Computational Approaches Can Be Used to Model the Reactions in Cells 524
Statistical Methods Are Critical For the Analysis of Biological Data 524
Summary 525
Problems 525
References 528
Chapter 9 Visualizing Cells 529
LOOKING AT CELLS IN THE LIGHT MICROSCOPE 529
The Light Microscope Can Resolve Details 0.2 μm Apart 530
Photon Noise Creates Additional Limits to Resolution When Light Levels Are Low 532
Living Cells Are Seen Clearly in a Phase-Contrast or a Differential-Interference-Contrast Microscope 533
Images Can Be Enhanced and Analyzed by Digital Techniques 534
Intact Tissues Are Usually Fixed and Sectioned Before Microscopy 535
Specific Molecules Can Be Located in Cells by Fluorescence Microscopy 536
Antibodies Can Be Used to Detect Specific Molecules 539
Imaging of Complex Three-Dimensional Objects Is Possible with the Optical Microscope 540
The Confocal Microscope Produces Optical Sections by Excluding Out-of-Focus Light 540
Individual Proteins Can Be Fluorescently Tagged in Living Cells and Organisms 542
Protein Dynamics Can Be Followed in Living Cells 543
Light-Emitting Indicators Can Measure Rapidly Changing Intracellular Ion Concentrations 546
Single Molecules Can Be Visualized by Total Internal Reflection Fluorescence Microscopy 547
Individual Molecules Can Be Touched, Imaged, and Moved Using Atomic Force Microscopy 548
Superresolution Fluorescence Techniques Can Overcome Diffraction-Limited Resolution 549
Superresolution Can Also be Achieved Using Single-Molecule Localization Methods 551
Summary 554
LOOKING AT CELLS AND MOLECULES IN THE ELECTRON MICROSCOPE 554
The Electron Microscope Resolves the Fine Structure of the Cell 554
Biological Specimens Require Special Preparation for Electron Microscopy 555
Specific Macromolecules Can Be Localized by Immunogold Electron Microscopy 556
Different Views of a Single Object Can Be Combined to Give a Three-Dimensional Reconstruction 557
Images of Surfaces Can Be Obtained by Scanning Electron Microscopy 558
Negative Staining and Cryoelectron Microscopy Both Allow Macromolecules to Be Viewed at High Resolution 559
Multiple Images Can Be Combined to Increase Resolution 561
Summary 562
Problems 563
References 564
Chapter 10 Membrane Structure 565
THE LIPID BILAYER 566
Phosphoglycerides, Sphingolipids, and Sterols Are the Major Lipids in Cell Membranes 566
Phospholipids Spontaneously Form Bilayers 568
The Lipid Bilayer Is a Two-dimensional Fluid 569
The Fluidity of a Lipid Bilayer Depends on Its Composition 571
Despite Their Fluidity, Lipid Bilayers Can Form Domains of Different Compositions 572
Lipid Droplets Are Surrounded by a Phospholipid Monolayer 573
The Asymmetry of the Lipid Bilayer Is Functionally Important 573
Glycolipids Are Found on the Surface of All Eukaryotic Plasma Membranes 575
Summary 576
MEMBRANE PROTEINS 576
Membrane Proteins Can Be Associated with the Lipid Bilayer in Various Ways 576
Lipid Anchors Control the Membrane Localization of Some Signaling Proteins 577
In Most Transmembrane Proteins, the Polypeptide Chain Crosses the Lipid Bilayer in an α-Helical Conformation 579
Transmembrane α Helices Often Interact with One Another 580
Some β Barrels Form Large Channels 580
Many Membrane Proteins Are Glycosylated 582
Membrane Proteins Can Be Solubilized and Purified in Detergents 583
Bacteriorhodopsin Is a Light-driven Proton (H+) Pump That Traverses the Lipid Bilayer as Seven α Helices 586
Membrane Proteins Often Function as Large Complexes 588
Many Membrane Proteins Diffuse in the Plane of the Membrane 588
Cells Can Confine Proteins and Lipids to Specific Domains Within a Membrane 590
The Cortical Cytoskeleton Gives Membranes Mechanical Strength and Restricts Membrane Protein Diffusion 591
Membrane-bending Proteins Deform Bilayers 593
Summary 594
Problems 595
References 596
Chapter 11 Membrane Transport of Small Molecules and the Electrical Properties of Membranes 597
PRINCIPLES OF MEMBRANE TRANSPORT 597
Protein-Free Lipid Bilayers Are Impermeable to Ions 598
There Are Two Main Classes of Membrane Transport Proteins:Transporters and Channels 598
Active Transport Is Mediated by Transporters Coupled to an Energy Source 599
Summary 600
TRANSPORTERS AND ACTIVE MEMBRANE TRANSPORT 600
Active Transport Can Be Driven by Ion-Concentration Gradients 601
Transporters in the Plasma Membrane Regulate Cytosolic pH 604
An Asymmetric Distribution of Transporters in Epithelial Cells Underlies the Transcellular Transport of Solutes 605
There Are Three Classes of ATP-Driven Pumps 606
A P-type ATPase Pumps Ca2+ into the Sarcoplasmic Reticulum in Muscle Cells 606
The Plasma Membrane Na+-K+ Pump Establishes Na+ and K+ Gradients Across the Plasma Membrane 607
ABC Transporters Constitute the Largest Family of Membrane Transport Proteins 609
Summary 611
CHANNELS AND THE ELECTRICAL PROPERTIES OF MEMBRANES 611
Aquaporins Are Permeable to Water But Impermeable to Ions 612
Ion Channels Are Ion-Selective and Fluctuate Between Open and Closed States 613
The Membrane Potential in Animal Cells Depends Mainly on K+ Leak Channels and the K+ Gradient Across the Plasma Membrane 615
The Resting Potential Decays Only Slowly When the Na+-K+ Pump Is Stopped 615
The Three-Dimensional Structure of a Bacterial K+ Channel Shows How an Ion Channel Can Work 617
Mechanosensitive Channels Protect Bacterial Cells Against Extreme Osmotic Pressures 619
The Function of a Neuron Depends on Its Elongated Structure 620
Voltage-Gated Cation Channels Generate Action Potentials in Electrically Excitable Cells 621
The Use of Channel rhodopsins Has Revolutionized the Study of Neural Circuits 623
Myelination Increases the Speed and Efficiency of Action Potential Propagation in Nerve Cells 625
Patch-Clamp Recording Indicates That Individual Ion Channels Open in an All-or-Nothing Fashion 626
Voltage-Gated Cation Channels Are Evolutionarily and Structurally Related 626
Different Neuron Types Display Characteristic Stable Firing Properties 627
Transmitter-Gated Ion Channels Convert Chemical Signals into Electrical Ones at Chemical Synapses 627
Chemical Synapses Can Be Excitatory or Inhibitory 629
The Acetylcholine Receptors at the Neuromuscular Junction Are Excitatory Transmitter-Gated Cation Channels 630
Neurons Contain Many Types of Transmitter-Gated Channels 631
Many Psychoactive Drugs Act at Synapses 631
Neuromuscular Transmission Involves the Sequential Activation of Five Different Sets of Ion Channels 632
Single Neurons Are Complex Computation Devices 633
Neuronal Computation Requires a Combination of at Least Three Kinds of K+ Channels 634
Long-Term Potentiation (LTP) in the Mammalian Hippocampus Depends on Ca2+ Entry Through NMDA-Receptor Channels 636
Summary 637
Problems 638
References 640
Chapter 12 Intracellular Compartments and Protein Sorting 641
THE COMPARTMENTALIZATION OF CELLS 641
All Eukaryotic Cells Have the Same Basic Set of Membrane-enclosed Organelles 641
Evolutionary Origins May Help Explain the Topological Relationships of Organelles 643
Proteins Can Move Between Compartments in Different Ways 645
Signal Sequences and Sorting Receptors Direct Proteins to the Correct Cell Address 647
Most Organelles Cannot Be Constructed De Novo: They Require Information in the Organelle Itself 648
Summary 649
THE TRANSPORT OF MOLECULES BETWEEN THE NUCLEUS AND THE CYTOSOL 649
Nuclear Pore Complexes Perforate the Nuclear Envelope 649
Nuclear Localization Signals Direct Nuclear Proteins to the Nucleus 650
Nuclear Import Receptors Bind to Both Nuclear Localization Signals and NPC Proteins 652
Nuclear Export Works Like Nuclear Import, But in Reverse 652
The Ran GTPase Imposes Directionality on Transport Through NPCs 653
Transport Through NPCs Can Be Regulated by Controlling Access to the Transport Machinery 654
During Mitosis the Nuclear Envelope Disassembles 656
Summary 657
THE TRANSPORT OF PROTEINS INTO MITOCHONDRIA AND CHLOROPLASTS 658
Translocation into Mitochondria Depends on Signal Sequences and Protein Translocators 659
Mitochondrial Precursor Proteins Are Imported as Unfolded Polypeptide Chains 660
ATP Hydrolysis and a Membrane Potential Drive Protein Import Into the Matrix Space 661
Bacteria and Mitochondria Use Similar Mechanisms to Insert Porins into their Outer Membrane 662
Transport Into the Inner Mitochondrial Membrane and Intermembrane Space Occurs Via Several Routes 663
Two Signal Sequences Direct Proteins to the Thylakoid Membrane in Chloroplasts 664
Summary 666
PEROXISOMES 666
Peroxisomes Use Molecular Oxygen and Hydrogen Peroxide to Perform Oxidation Reactions 666
A Short Signal Sequence Directs the Import of Proteins into Peroxisomes 667
Summary 669
THE ENDOPLASMIC RETICULUM 669
The ER Is Structurally and Functionally Diverse 670
Signal Sequences Were First Discovered in Proteins Imported into the Rough ER 672
A Signal-Recognition Particle (SRP) Directs the ER Signal Sequence to a Specific Receptor in the Rough ER Membrane 673
The Polypeptide Chain Passes Through an Aqueous Channel in the Translocator 675
Translocation Across the ER Membrane Does Not Always Require Ongoing Polypeptide Chain Elongation 677
In Single-Pass Transmembrane Proteins, a Single Internal ER Signal Sequence Remains in the Lipid Bilayer as a Membrane-spanning α Helix 677
Combinations of Start-Transfer and Stop-Transfer Signals Determine the Topology of Multipass Transmembrane Proteins 679
ER Tail-anchored Proteins Are Integrated into the ER Membrane by a Special Mechanism 682
Translocated Polypeptide Chains Fold and Assemble in the Lumen of the Rough ER 682
Most Proteins Synthesized in the Rough ER Are Glycosylated by the Addition of a Common N-Linked Oligosaccharide 683
Oligosaccharides Are Used as Tags to Mark the State of Protein Folding 685
Improperly Folded Proteins Are Exported from the ER and Degraded in the Cytosol 685
Misfolded Proteins in the ER Activate an Unfolded Protein Response 686
Some Membrane Proteins Acquire a Covalently Attached Glycosylphosphatidylinositol (GPI) Anchor 688
The ER Assembles Most Lipid Bilayers 689
Summary 691
Problems 692
References 694
Chapter 13 Intracellular Membrane Traffic 695
THE MOLECULAR MECHANISMS OF MEMBRANE TRANSPORT AND THE MAINTENANCE OF COMPARTMENTAL DIVERSITY 697
There Are Various Types of Coated Vesicles 697
The Assembly of a Clathrin Coat Drives Vesicle Formation 697
Adaptor Proteins Select Cargo into Clathrin-Coated Vesicles 698
Phosphoinositides Mark Organelles and Membrane Domains 700
Membrane-Bending Proteins Help Deform the Membrane During Vesicle Formation 701
Cytoplasmic Proteins Regulate the Pinching-Off and Uncoating of Coated Vesicles 701
Monomeric GTPases Control Coat Assembly 703
Not All Transport Vesicles Are Spherical 704
Rab Proteins Guide Transport Vesicles to Their Target Membrane 705
Rab Cascades Can Change the Identity of an Organelle 707
SNAREs Mediate Membrane Fusion 708
Interacting SNAREs Need to Be Pried Apart Before They Can Function Again 709
Summary 710
TRANSPORT FROM THE ER THROUGH THE GOLGI APPARATUS 710
Proteins Leave the ER in COPII-Coated Transport Vesicles 711
Only Proteins That Are Properly Folded and Assembled Can Leave the ER 712
Vesicular Tubular Clusters Mediate Transport from the ER to the Golgi Apparatus 712
The Retrieval Pathway to the ER Uses Sorting Signals 713
Many Proteins Are Selectively Retained in the Compartments in Which They Function 714
The Golgi Apparatus Consists of an Ordered Series of Compartments 715
Oligosaccharide Chains Are Processed in the Golgi Apparatus 716
Proteoglycans Are Assembled in the Golgi Apparatus 718
What Is the Purpose of Glycosylation? 719
Transport Through the Golgi Apparatus May Occur by Cisternal Maturation 720
Golgi Matrix Proteins Help Organize the Stack 721
Summary 722
TRANSPORT FROM THE TRANS GOLGI NETWORK TO LYSOSOMES 722
Lysosomes Are the Principal Sites of Intracellular Digestion 722
Lysosomes Are Heterogeneous 723
Plant and Fungal Vacuoles Are Remarkably Versatile Lysosomes 724
Multiple Pathways Deliver Materials to Lysosomes 725
Autophagy Degrades Unwanted Proteins and Organelles 726
A Mannose 6-Phosphate Receptor Sorts Lysosomal Hydrolases in the Trans Golgi Network 727
Defects in the GIcNAc Phosphotransferase Cause a Lysosomal Storage Disease in Humans 728
Some Lysosomes and Multivesicular Bodies Undergo Exocytosis 729
Summary 729
TRANSPORT INTO THE CELL FROM THE PLASMA MEMBRANE: ENDOCYTOSIS 730
Pinocytic Vesicles Form from Coated Pits in the Plasma Membrane 731
Not All Pinocytic Vesicles Are Clathrin-Coated 731
Cells Use Receptor-Mediated Endocytosis to Import Selected Extracellular Macromolecules 732
Specific Proteins Are Retrieved from Early Endosomes and Returned to the Plasma Membrane 734
Plasma Membrane Signaling Receptors are Down-Regulated by Degradation in Lysosomes 735
Early Endosomes Mature into Late Endosomes 735
ESCRT Protein Complexes Mediate the Formation of Intralumenal Vesicles in Multivesicular Bodies 736
Recycling Endosomes Regulate Plasma Membrane Composition 737
Specialized Phagocytic Cells Can Ingest Large Particles 738
Summary 740
TRANSPORT FROM THE TRANS GOLGI NETWORK TO THE CELL EXTERIOR: EXOCYTOSIS 741
Many Proteins and Lipids Are Carried Automatically from the Trans Golgi Network (TGN) to the Cell Surface 741
Secretory Vesicles Bud from the Trans Golgi Network 742
Precursors of Secretory Proteins Are Proteolytically Processed During the Formation of Secretory Vesicles 743
Secretory Vesicles Wait Near the Plasma Membrane Until Signaled to Release Their Contents 744
For Rapid Exocytosis, Synaptic Vesicles Are Primed at the Presynaptic Plasma Membrane 744
Synaptic Vesicles Can Form Directly from Endocytic Vesicles 746
Secretory Vesicle Membrane Components Are Quickly Removed from the Plasma Membrane 746
Some Regulated Exocytosis Events Serve to Enlarge the Plasma Membrane 748
Polarized Cells Direct Proteins from the Trans Golgi Network to the Appropriate Domain of the Plasma Membrane 748
Summary 750
Problems 750
References 752
Chapter 14 Energy Conversion: Mitochondria and Chloroplasts 753
THE MITOCHONDRION 755
The Mitochondrion Has an Outer Membrane and an Inner Membrane 757
The Inner Membrane Cristae Contain the Machinery for Electron Transport and ATP Synthesis 758
The Citric Acid Cycle in the Matrix Produces NADH 758
Mitochondria Have Many Essential Roles in Cellular Metabolism 759
A Chemiosmotic Process Couples Oxidation Energy to ATP Production 761
The Energy Derived from Oxidation Is Stored as an Electrochemical Gradient 762
Summary 763
THE PROTON PUMPS OF THE ELECTRON-TRANSPORT CHAIN 763
The Redox Potential Is a Measure of Electron Affinities 763
Electron Transfers Release Large Amounts of Energy 764
Transition Metal Ions and Quinones Accept and Release Electrons Readily 764
NADH Transfers Its Electrons to Oxygen Through Three Large Enzyme Complexes Embedded in the Inner Membrane 766
The NADH Dehydrogenase Complex Contains Separate Modules for Electron Transport and Proton Pumping 768
Cytochrome c Reductase Takes Up and Releases Protons on the Opposite Side of the Crista Membrane, Thereby Pumping Protons 768
The Cytochrome c Oxidase Complex Pumps Protons and Reduces O2 Using a Catalytic Iron-Copper Center 770
The Respiratory Chain Forms a Supercomplex in the Crista Membrane 772
Protons Can Move Rapidly Through Proteins Along Predefined Pathways 773
Summary 774
ATP PRODUCTION IN MITOCHONDRIA 774
The Large Negative Value of AG for ATP Hydrolysis Makes ATP Useful to the Cell 774
The ATP Synthase Is a Nanomachine that Produces ATP by Rotary Catalysis 776
Proton-driven Turbines Are of Ancient Origin 777
Mitochondrial Cristae Help to Make ATP Synthesis Efficient 778
Special Transport Proteins Exchange ATP and ADP Through the Inner Membrane 779
Chemiosmotic Mechanisms First Arose in Bacteria 780
Summary 782
CHLOROPLASTS AND PHOTOSYNTHESIS 782
Chloroplasts Resemble Mitochondria But Have a Separate Thylakoid Compartment 782
Chloroplasts Capture Energy from Sunlight and Use It to Fix Carbon 783
Carbon Fixation Uses ATP and NADPH to Convert CO2 into Sugars 784
Sugars Generated by Carbon Fixation Can Be Stored as Starch or Consumed to Produce ATP 785
The Thylakoid Membranes of Chloroplasts Contain the Protein Complexes Required for Photosynthesis and ATP Generation 786
Chlorophyll-Protein Complexes Can Transfer Either Excitation Energy or Electrons 787
A Photosystem Consists of an Antenna Complex and a Reaction Center 788
The Thylakoid Membrane Contains Two Different Photosystems Working in Series 789
Photosystem Ⅱ Uses a Manganese Cluster to Withdraw Electrons From Water 790
The Cytochrome b6-f Complex Connects Photosystem Ⅱ to Photosystem Ⅰ 791
Photosystem Ⅰ Carries Out the Second Charge-Separation Step in the Z Scheme 792
The Chloroplast ATP Synthase Uses the Proton Gradient Generated by the Photosynthetic Light Reactions to Produce ATP 793
All Photosynthetic Reaction Centers Have Evolved From a Common Ancestor 793
The Proton-Motive Force for ATP Production in Mitochondria and Chloroplasts Is Essentially the Same 794
Chemiosmotic Mechanisms Evolved in Stages 794
By Providing an Inexhaustible Source of Reducing Power,Photosynthetic Bacteria Overcame a Major Evolutionary Obstacle 796
The Photosynthetic Electron-Transport Chains of Cyanobacteria Produced Atmospheric Oxygen and Permitted New Life-Forms 796
Summary 798
THE GENETIC SYSTEMS OF MITOCHONDRIA AND CHLOROPLASTS 800
The Genetic Systems of Mitochondria and Chloroplasts Resemble Those of Prokaryotes 800
Over Time, Mitochondria and Chloroplasts Have Exported Most of Their Genes to the Nucleus by Gene Transfer 801
The Fission and Fusion of Mitochondria Are Topologically Complex Processes 802
Animal Mitochondria Contain the Simplest Genetic Systems Known 803
Mitochondria Have a Relaxed Codon Usage and Can Have a Variant Genetic Code 804
Chloroplasts and Bacteria Share Many Striking Similarities 806
Organelle Genes Are Maternally Inherited in Animals and Plants 807
Mutations in Mitochondrial DNA Can Cause Severe Inherited Diseases 807
The Accumulation of Mitochondrial DNA Mutations Is a Contributor to Aging 808
Why Do Mitochondria and Chloroplasts Maintain a Costly Separate System for DNA Transcription and Translation? 808
Summary 809
Problems 809
References 811
Chapter 15 Cell Signaling 813
PRINCIPLES OF CELL SIGNALING 813
Extracellular Signals Can Act Over Short or Long Distances 814
Extracellular Signal Molecules Bind to Specific Receptors 815
Each Cell Is Programmed to Respond to Specific Combinations of Extracellular Signals 816
There Are Three Major Classes of Cell-Surface Receptor Proteins 818
Cell-Surface Receptors Relay Signals Via Intracellular Signaling Molecules 819
Intracellular Signals Must Be Specific and Precise in a Noisy Cytoplasm 820
Intracellular Signaling Complexes Form at Activated Receptors 822
Modular Interaction Domains Mediate Interactions Between Intracellular Signaling Proteins 822
The Relationship Between Signal and Response Varies in Different Signaling Pathways 824
The Speed of a Response Depends on the Turnover of Signaling Molecules 825
Cells Can Respond Abruptly to a Gradually Increasing Signal 827
Positive Feedback Can Generate an All-or-None Response 828
Negative Feedback is a Common Motif in Signaling Systems 829
Cells Can Adjust Their Sensitivity to a Signal 830
Summary 831
SIGNALING THROUGH G-PROTEIN-COUPLED RECEPTORS 832
Trimeric G Proteins Relay Signals From GPCRs 832
Some G Proteins Regulate the Production of Cyclic AMP 833
Cyclic-AMP-Dependent Protein Kinase (PKA) Mediates Most of the Effects of Cyclic AMP 834
Some G Proteins Signal Via Phospholipids 836
Ca2+ Functions as a Ubiquitous Intracellular Mediator 838
Feedback Generates Ca2+ Waves and Oscillations 838
Ca2+/Calmodulin-Dependent Protein Kinases Mediate Many Responses to Ca2+ Signals 840
Some G Proteins Directly Regulate Ion Channels 843
Smell and Vision Depend on GPCRs That Regulate Ion Channels 843
Nitric Oxide Is a Gaseous Signaling Mediator That Passes Between Cells 846
Second Messengers and Enzymatic Cascades Amplify Signals 848
GPCR Desensitization Depends on Receptor Phosphorylation 848
Summary 849
SIGNALING THROUGH ENZYME-COUPLED RECEPTORS 850
Activated Receptor Tyrosine Kinases (RTKs) Phosphorylate Themselves 850
Phosphorylated Tyrosines on RTKs Serve as Docking Sites for Intracellular Signaling Proteins 852
Proteins with SH2 Domains Bind to Phosphorylated Tyrosines 852
The GTPase Ras Mediates Signaling by Most RTKs 854
Ras Activates a MAP Kinase Signaling Module 855
Scaffold Proteins Help Prevent Cross-talk Between Parallel MAP Kinase Modules 857
Rho Family GTPases Functionally Couple Cell-Surface Receptors to the Cytoskeleton 858
PI 3-Kinase Produces Lipid Docking Sites in the Plasma Membrane 859
The PI-3-Kinase-Akt Signaling Pathway Stimulates Animal Cells to Survive and Grow 860
RTKs and GPCRs Activate Overlapping Signaling Pathways 861
Some Enzyme-Coupled Receptors Associate with Cytoplasmic Tyrosine Kinases 862
Cytokine Receptors Activate the JAK-STAT Signaling Pathway 863
Protein Tyrosine Phosphatases Reverse Tyrosine Phosphorylations 864
Signal Proteins of the TGFβ Superfamily Act Through Receptor Seri ne/Threonine Kinases and Smads 865
Summary 866
ALTERNATIVE SIGNALING ROUTES IN GENE REGULATION 867
The Receptor Notch Is a Latent Transcription Regulatory Protein 867
Wnt Proteins Bind to Frizzled Receptors and Inhibit the Degradation of β-Catenin 868
Hedgehog Proteins Bind to Patched, Relieving Its Inhibition of Smoothened 871
Many Stressful and Inflammatory Stimuli Act Through an NFkB-Dependent Signaling Pathway 873
Nuclear Receptors Are Ligand-Modulated Transcription Regulators 874
Circadian Clocks Contain Negative Feedback Loops That Control Gene Expression 876
Three Proteins in a Test Tube Can Reconstitute a Cyanobacterial Circadian Clock 878
Summary 879
SIGNALING IN PLANTS 880
Multicellularity and Cell Communication Evolved Independently in Plants and Animals 880
Receptor Serine/Threonine Kinases Are the Largest Class of Cell-Surface Receptors in Plants 881
Ethylene Blocks the Degradation of Specific Transcription Regulatory Proteins in the Nucleus 881
Regulated Positioning of Auxin Transporters Patterns Plant Growth 882
Phytochromes Detect Red Light, and Cryptochromes Detect Blue Light 883
Summary 885
Problems 886
References 887
Chapter 16 The Cytoskeleton 889
FUNCTION AND ORIGIN OF THE CYTOSKELETON 889
Cytoskeletal Filaments Adapt to Form Dynamic or Stable Structures 890
The Cytoskeleton Determines Cellular Organization and Polarity 892
Filaments Assemble from Protein Subunits That Impart Specific Physical and Dynamic Properties 893
Accessory Proteins and Motors Regulate Cytoskeletal Filaments 894
Bacterial Cell Organization and Division Depend on Homologs of Eukaryotic Cytoskeletal Proteins 896
Summary 898
ACTIN AND ACTIN-BINDING PROTEINS 898
Actin Subunits Assemble Head-to-Tail to Create Flexible, Polar Filaments 898
Nucleation Is the Rate-Limiting Step in the Formation of Actin Filaments 899
Actin Filaments Have Two Distinct Ends That Grow at Different Rates 900
ATP Hydrolysis Within Actin Filaments Leads to Treadmilling at Steady State 901
The Functions of Actin Filaments Are Inhibited by Both Polymer-stabilizing and Polymer-destabilizing Chemicals 904
Actin-Binding Proteins Influence Filament Dynamics and Organization 904
Monomer Availability Controls Actin Filament Assembly 906
Actin-Nucleating Factors Accelerate Polymerization and Generate Branched or Straight Filaments 906
Actin-Filament-Binding Proteins Alter Filament Dynamics 907
Severing Proteins Regulate Actin Filament Depolymerization 909
Higher-Order Actin Filament Arrays Influence Cellular Mechanical Properties and Signaling 911
Bacteria Can Hijack the Host Actin Cytoskeleton 913
Summary 914
MYOSIN AND ACTIN 915
Actin-Based Motor Proteins Are Members of the Myosin Superfamily 915
Myosin Generates Force by Coupling ATP Hydrolysis to Conformational Changes 916
Sliding of Myosin II Along Actin Filaments Causes Muscles to Contract 916
A Sudden Rise in Cytosolic Ca2+Concentration Initiates Muscle Contraction 920
Heart Muscle Is a Precisely Engineered Machine 923
Actin and Myosin Perform a Variety of Functions in Non-Muscle Cells 923
Summary 925
MICROTUBULES 925
Microtubules Are Hollow Tubes Made of Protofilaments 926
Microtubules Undergo Dynamic Instability 927
Microtubule Functions Are Inhibited by Both Polymer-stabilizing and Polymer-destabilizing Drugs 929
A Protein Complex Containing γ-Tubulin Nucleates Microtubules 929
Microtubules Emanate from the Centrosome in Animal Cells 930
Microtubule-Binding Proteins Modulate Filament Dynamics and Organization 932
Microtubule Plus-End-Binding Proteins Modulate Microtubule Dynamics and Attachments 932
Tubulin-Sequestering and Microtubule-Severing Proteins Destabilize Microtubules 935
Two Types of Motor Proteins Move Along Microtubules 936
Microtubules and Motors Move Organelles and Vesicles 938
Construction of Complex Microtubule Assemblies Requires Microtubule Dynamics and Motor Proteins 940
Motile Cilia and Flagella Are Built from Microtubules and Dyneins 941
Primary Cilia Perform Important Signaling Functions in Animal Cells 942
Summary 943
INTERMEDIATE FILAMENTS AND SEPTINS 944
Intermediate Filament Structure Depends on the Lateral Bundling and Twisting of Coiled-Coils 945
Intermediate Filaments Impart Mechanical Stability to Animal Cells 946
Linker Proteins Connect Cytoskeletal Filaments and Bridge the Nuclear Envelope 948
Septins Form Filaments That Regulate Cell Polarity 949
Summary 950
CELL POLARIZATION AND MIGRATION 951
Many Cells Can Crawl Across a Solid Substratum 951
Actin Polymerization Drives Plasma Membrane Protrusion 951
Lamellipodia Contain All of the Machinery Required for Cell Motility 953
Myosin Contraction and Cell Adhesion Allow Cells to Pull Themselves Forward 954
Cell Polarization Is Controlled by Members of the Rho Protein Family 955
Extracellular Signals Can Activate the Three Rho Protein Family Members 958
External Signals Can Dictate the Direction of Cell Migration 958
Communication Among Cytoskeletal Elements Coordinates Whole-Cell Polarization and Locomotion 959
Summary 960
Problems 960
References 962
Chapter 17 The Cell Cycle 963
OVERVIEW OF THE CELL